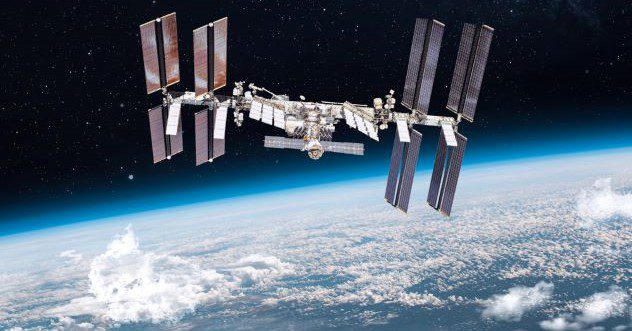
The machines we’ve built throughout history are not just tools; they are the embodiment of human intelligence and ambition. The complexity of these creations often mirrors the complexity of the human mind itself. From probing the depths of space to unlocking the mysteries of the atom, our most intricate machines have enabled us to expand the frontiers of science and technology.
They are marvels of innovation, each a testament to the relentless human pursuit of knowledge and mastery over the elements. These devices, sophisticated beyond their years, have paved the way for new discoveries and continue to inspire awe and wonder.
Related: 10 Ancient Technologies We Cannot Recreate Today
10 Quantum Computers
Quantum computing is like stepping into a world where computers have superpowers. Instead of flipping switches that are either off or on (like the 0s and 1s in regular computers), quantum computers use special particles that can be in a sort of “super-switch” state where they’re both off and on at the same time. This is possible thanks to two mind-bending features of quantum mechanics called “superposition” and “entanglement.”
Superposition lets these special particles, called qubits, do many calculations at once instead of one by one. Imagine you’re trying to solve a maze, and instead of one version of you walking down each path in turn, you could have many versions exploring all paths simultaneously. That’s how a quantum computer approaches problem solving, which makes it incredibly fast.
Entanglement is another quantum trick. If you entangle two qubits, they become like magic twins—what happens to one instantly affects the other, no matter how far apart they are. This helps quantum computers link up their super-switches in powerful ways to solve complex problems that would take normal computers ages to work through.
But quantum computers are a bit like race cars—they’re super fast but also very tricky to handle. They’re sensitive to the smallest disturbances, like a bump in the road or a change in weather, which can throw off their calculations. That’s why they need special conditions, like extreme cold or vacuum chambers, to work properly.
Quantum computers are currently in development, not yet capable of replacing conventional computers. However, they hold the potential for future advancements, such as creating new medicines, enhancing electric car batteries, and optimizing airplane efficiency. Despite them not being ready, their possibilities are immense. Similar to the early computers, quantum computers have the potential to bring about significant global changes.[1]
9 The Tokamak Fusion Test Reactor
The Tokamak Fusion Test Reactor (TFTR) was a groundbreaking project at the Princeton Plasma Physics Laboratory, operational from 1982 to 1997. It was a pioneer in fusion research, achieving plasma temperatures of 510 million degrees centigrade, a feat that far surpasses the 100 million degrees required for the kind of fusion that could one day power our cities.
In a landmark experiment in 1993, the TFTR used a mixture of deuterium and tritium—two isotopes of hydrogen—as fuel. This mixture is key to a practical fusion reactor, the kind that could realistically supply energy to our power grids. The following year, the reactor produced an unprecedented 10.7 million watts of fusion power, demonstrating the potential to provide electricity to thousands of households.
The TFTR also explored innovative ways to improve plasma confinement, which is crucial for maintaining the conditions necessary for fusion. In 1995, they experimented with a technique known as enhanced reversed shear, which adjusted the magnetic fields to significantly lower the turbulence within the plasma, aiding in its stability.
The achievements of the TFTR have been instrumental in advancing our understanding of fusion energy, bringing us closer to harnessing this clean and abundant energy source. The reactor not only met its scientific objectives but also excelled in its hardware performance, contributing valuable insights to the field of fusion technology.[2]
8 Z Machine
The Z Machine in Albuquerque, New Mexico, housed within the Sandia National Laboratories, is a marvel of modern science, holding the title of the most powerful and efficient laboratory radiation source in the world. It’s capable of producing conditions not found anywhere else on Earth, replicating the dense plasma that exists within white dwarf stars.
When activated, the Z Machine directs a staggering 20 million amps of electricity—over a thousand times more powerful than a lightning bolt—toward a tiny target. This target contains a hohlraum, a small metal container with hundreds of tungsten wires finer than human hair. These wires are transformed into plasma, the same material that constitutes stars, allowing researchers to study “star stuff” right here on our planet.
The origins of the Z Machine trace back to the 1970s when the Department of Energy sought to simulate the fusion reactions of thermonuclear bombs in a controlled laboratory setting. This led to the development of the Z Pulsed Power Facility, or the Z Machine, in 1996. The science behind it involves complex concepts like Z-pinch, Lorentz forces, plasma compression, and magnetohydrodynamic (MHD) instability.
The Z Machine’s experiments contribute to various scientific fields, including weapons research, where it provides data for computer models of nuclear weapons, aiding in the assessment of the U.S. nuclear stockpile’s reliability and safety. It’s also a beacon of hope in the quest for fusion energy, showing promise in generating more energy than what is inputted, a significant step towards sustainable fusion power.
Additionally, the Z Machine’s research extends to understanding the cosmos, shedding light on star formation and the activities at their cores. It’s even challenging existing theories about the ions in black holes’ accretion discs. Despite its significance, the Z Machine is not open to the public, and access to the Sandia National Laboratories involves navigating through considerable bureaucracy.[3]
7 Antikythera Mechanism
The Antikythera mechanism, an ancient Greek device discovered in a shipwreck near the island of Antikythera in 1900, dates back to between 60 and 70 BC. Remarkably complex, it served as an astronomical calculator far ahead of its time. Its intricate gearwheels indicate that ancient Greek technology was more advanced than previously assumed.
The Antikythera mechanism could predict astronomical positions and eclipses for calendrical and astrological purposes. It drew on theories and knowledge from Babylonian astronomy and used a sophisticated understanding of lunar and solar cycles. The design incorporated period relations known from Babylonian records to predict celestial events with incredible accuracy.
Recent studies by the UCL Antikythera Research Team shed new light on the mechanism’s functions and offered a fresh understanding of the gearing on the device’s front. These insights led to a greater appreciation of the mechanism’s sophistication, suggesting that the ancient Greeks possessed capabilities that challenge our assumptions about their technological advancements.
The mechanism also reflects the Greeks’ understanding of the geocentric model of the universe, where Earth was believed to be at the center, and the “fixed stars” and “wanderers” (planets) moved in intricate patterns in the sky. The mechanism tracked these movements and predicted their positions with its gear trains, calibrated to known astronomical cycles.[4]
6 James Webb Space Telescope
The James Webb Space Telescope (JWST) represents one of NASA’s most ambitious and technically challenging projects to date. It’s an unprecedented infrared observatory designed to provide a deeper view of the cosmos than any previous telescope. The development of the JWST required the collective expertise of hundreds of scientists, engineers, and optics specialists, along with the collaboration of three major space agencies: NASA, the European Space Agency (ESA), and the Canadian Space Agency (CSA). Over 1,200 individuals worldwide have contributed to bringing this powerful space telescope to fruition.
The JWST’s design process was extensive and involved the creation of ten new technological innovations, termed “enabling technologies,” which were essential for its construction. These advancements will allow the JWST to surpass the capabilities of its predecessor, the Hubble Space Telescope, by nearly 100 times. The JWST is expected to provide invaluable insights into the origins of the universe, the formation of stars and planets, and detailed analysis of planetary bodies within and beyond our solar system.
The engineering challenges of the telescope were immense, requiring it to be both large and capable of operating at very cold temperatures in space. It was designed to fold up for its journey into space and then unfold remotely once in orbit. This required the components to be built in a way that would compensate for the lack of gravity and the vacuum of space.
To ensure the telescope’s readiness for space, NASA subjected it to rigorous testing, including exposure to extreme temperatures in a massive cryogenic chamber known as “Chamber A” in Houston, Texas. The telescope also underwent a series of structural tests to simulate the conditions of launch and the harsh environment of space.[5]
5 International Thermonuclear Experimental Reactor (ITER)
The ITER project is a large-scale scientific experiment that aims to demonstrate the feasibility of fusion as a large-scale and carbon-free source of energy based on the same principle that powers our Sun and stars. The project is a collaboration of 35 nations and is currently under construction in Southern France. In a fusion process, energy is released when the nuclei of two light atoms fuse together to form a heavier nucleus.
To achieve this on Earth, the fuel (typically isotopes of hydrogen) must be heated to temperatures over 150 million degrees Celsius, forming a hot plasma. Strong magnetic fields are used to keep this plasma away from the reactor’s walls so that it doesn’t cool down and lose its energy potential. The goal of ITER is not to produce electricity but to prove that fusion can be harnessed to generate power. If successful, it could pave the way for fusion reactors that provide a virtually limitless supply of energy without the carbon emissions associated with current energy sources.
Despite the initial ambition, ITER is now billions of dollars over budget and decades behind schedule, with the latest official cost estimate exceeding €20 billion ($22 billion). The project, which began in 2006 with an estimated €5 billion budget and a 10-year completion plan, is now grappling with technical setbacks and regulatory issues that threaten to further delay its completion.
Several factors have contributed to the delays and cost overruns. Key components of the reactor have arrived late and with defects, such as thermal shields that cracked due to improper welding and parts of the vacuum vessel that did not meet the required precision. The French Nuclear Safety Authority has also halted assembly over concerns about radiation shielding, demanding more robust safety measures.
The situation raises questions about the feasibility of such large-scale international scientific projects and whether the potential benefits of fusion energy will justify the escalating costs and delays. The ITER project’s struggles reflect the inherent challenges of pioneering complex technology and the difficulties of international collaboration on such an ambitious scale.[6]
4 Deepwater Horizon
The Deepwater Horizon was a semi-submersible platform capable of drilling in ultra-deep waters, designed to operate under challenging surface conditions at depths reaching up to 10,000 feet (3,048 meters), manned by a crew of 135 specialists.
Unlike fixed-position vessels, this rig maintained its location over the well using dynamic positioning systems, including thrusters and propellers, allowing it to adjust its position as needed. The design of such semi-submersible platforms incorporates ballasted pontoons, enhancing their stability against waves and providing steadiness superior to conventional boats. Despite their robust structure, these platforms are not characterized by large deck areas, but they are equipped with essential control and operation centers, helipads, and cargo areas.
The Deepwater Horizon disaster, which began with an explosion on April 20, 2010, marked the most notorious offshore disaster in recent memory. The rig, valued at over $560 million, was drilling in the Macondo project offshore from Louisiana when it met with disaster. The explosion resulted in 11 missing and presumed dead workers and approximately 17 injured. The subsequent sinking of the rig led to a massive oil spill, with initial reports of a five-mile (8-kilometer) long slick. Efforts to contain the spill were monumental, involving BP and U.S. authorities attempting to activate a failed blowout preventer and employing various technologies to stop the oil flow.
The spill posed a significant threat to the fragile ecosystems and wildlife off the Louisiana coast. The U.S. Coast Guard’s initial estimate of the leak was 1,000 barrels per day, which was later revised to a staggering 5,000 barrels per day. This prompted a series of responses, including controlled burns of the oil slick, a declaration of a state of emergency by Louisiana, and a halt to new drilling as ordered by President Obama until the cause of the accident was determined.[7]
3 Apollo Guidance Computer (AGC)
Despite the common belief that modern devices surpass the technology of the past, the Apollo computer holds its own as an engineering marvel. It was a key player in the success of the moon landing, handling complex calculations and controlling the spacecraft’s components, which was beyond human capacity. Margaret Hamilton led a team of 350 to develop the software for the mission, which was advanced for its time, allowing multiple operations to run simultaneously in a very limited memory space.
Hamilton’s team’s software ingenuity was instrumental in averting a system overload that could have jeopardized the moon landing, solidifying her legacy in computer science and software engineering. The computer’s interface was unique, using “verb” and “noun” codes for astronauts to communicate with it. Notably, during the Apollo 11 mission, the computer’s software, designed by J. Halcombe Laning, prioritized tasks in a way that saved the mission from failure due to faulty data.
The AGC was the computational core that kept the Apollo missions on track, digesting and acting upon vast quantities of navigational data to avert deviation from the intended path. It utilized a combination of read-only memory (ROM) for unchangeable tasks and random-access memory (RAM) for variable operations, enabling it to juggle numerous tasks at once.
This was particularly vital during critical phases of the mission, like spacecraft rendezvous and docking. The AGC’s flawless performance is a hallmark of what can be achieved at the intersection of human creativity and technological prowess, a legacy that continues to inspire awe and drive innovation in space exploration.[8]
2 International Space Station (ISS)
The International Space Station (ISS) works as a service station for satellites and as a launch base for missions beyond Earth’s orbit. The ISS was designed to provide a pristine zero-gravity environment for a wide range of experiments, which poses significant architectural challenges and has increased the cost and complexity of the project.
Launched into orbit in 1998, it has been continuously occupied since November 2, 2000. The ISS is a collaborative effort among multiple countries, with major contributions from the United States, Russia, and the European Space Agency, as well as Canada and Japan. It serves as a microgravity and space environment research laboratory where scientific research is conducted in astrobiology, astronomy, meteorology, physics, and other fields.
The ISS orbits Earth at an altitude of approximately 250 miles (402 kilometers) and is visible to the naked eye. It’s as large as a football field, including the end zones, and has a mass of about 925,335 pounds (419,725 kilograms) without visiting vehicles. The station has been visited by 258 individuals from 20 countries, with the United States and Russia being the top participating countries.
Astronauts typically spend around six months on the ISS, conducting experiments, performing spacewalks, and engaging in outreach activities. Life on the ISS includes conducting research that’s vital for future long-term space exploration, such as to the Moon or Mars. The effects of microgravity on human health are a significant area of study, with changes observed in muscles, bones, the cardiovascular system, and eyesight.[9]
1 Large Hadron Collider (LHC)
The Large Hadron Collider (LHC) at CERN is the world’s largest and most powerful particle accelerator. Operational since September 10, 2008, it is a central piece of CERN’s accelerator complex. The LHC is a 16.7-mile (27-kilometer) ring of superconducting magnets, accompanied by accelerating structures that boost the energy of particles as they travel through it.
Within this massive structure, two high-energy particle beams are propelled to near-light speeds and directed to collide with each other. These beams move in opposite directions within separate vacuum tubes, guided by the magnetic field of superconducting electromagnets. These magnets require cooling to -271.3°C, colder than outer space, achieved through a liquid helium distribution system.
The LHC uses thousands of magnets of various types and sizes to manipulate the particle beams. This includes 1,232 dipole magnets to bend the beams and 392 quadrupole magnets to focus them. Before collision, special magnets “squeeze” the particles closer to increase collision chances, a task compared to trying to make two needles—6.2 miles (10 kilometers apart)—meet precisely halfway.
The LHC aims to answer key questions in physics, such as the origin of mass, the search for supersymmetry, the nature of dark matter and dark energy, the imbalance between matter and antimatter, and the properties of the quark-gluon plasma. It was conceived in the 1980s, approved in 1994, and has had significant milestones, including the discovery of the Higgs boson in 2012.
It generates over 30 petabytes of data annually, stored and archived at CERN’s Data Centre. The construction costs totaled approximately 4.3 billion CHF, with ongoing operational costs forming a significant part of CERN’s budget. The LHC’s power consumption is substantial, with estimates of around 750 GWh per year.[10]